Tracing the History of Polymeric Materials, Part 26: High-Performance Thermoplastics
The majority of the polymers that today we rely on for outstanding performance — such as polysulfone, polyethersulfone, polyphenylsulfone and PPS — were introduced in the period between 1965 and 1985. Here’s how they entered your toolbox of engineering of materials.
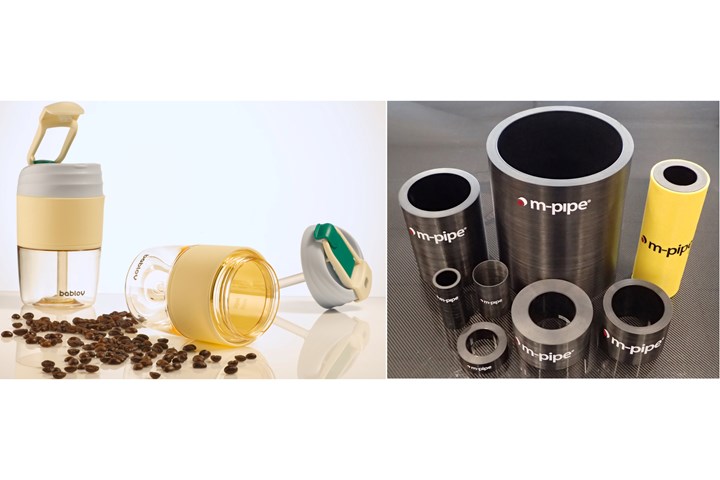
Two decades of extensive chemical innovation (1965-85) brought new high-performance engineering materials such as BASF’s Ultrason polysulfone in the mug at left and Victrex’s PEEK in the undersea oil/gas pipe at right. (Photos: BASF, Victrex)
As the development of new polymers moved into the so-called modern era, the history of these developments becomes more prosaic and the stories are less colorful and not as well documented. This is probably due to movement of the research from creative and inspired individuals working independently to large chemical companies with well-structured R&D programs. At the same time, polymers developed after 1965 tend to be those that we associate with high performance.
Not all high-performance polymers came late in the historical development. As was previously mentioned, polyamide-imide, commercially known as Torlon, was first developed at DuPont in the mid-1950s before being commercialized by Amoco in the early 1960s. (Today, it’s a product of Solvay.) And nylon, first created in the 1930’s, can arguably be viewed as the first engineering thermoplastic. But the majority of the polymers that we rely on today for outstanding performance were introduced in the period between 1965 and 1985.
Union Carbide commercialized polysulfone under the trade name Udel in 1965. Imperial Chemical Industries (ICI) and 3M also filed patents for this chemistry at about the same time. This elevated the upper limit of the glass -ransition temperature (Tg) for amorphous polymers from 150-155°C for polycarbonate to 185-190°C while also introducing a high degree of hydrolysis resistance. Polyethersulfone and polyphenylsulfone, developed in the early to mid-1970s, extended the thermal capabilities of high-performance amorphous thermoplastics to 215-220°C. Of course, Torlon exceeded all of these materials in heat resistance. But the challenges associated with processing and the need for extended post-bake routines to achieve optimal performance kept this a niche material.
Polyphenylene sulfide (PPS) was first produced in 1967 by Edmonds and Hill while they were working at Philips Petroleum, reacting dichlorobenzene with sodium sulfide, as shown in the accompanying figure. Commercialized as Ryton, the initial grades were based on a branched polymer and relied on relatively high levels of fillers and reinforcements. Later grades were based on a linear polymer that also offered a lighter natural color. PPS was the first of several high-performance semi-crystalline polymers that required the use of mold temperatures higher than those achievable with hot water to achieve an optimal level of crystallization.
This was not well understood when PPS was first developed and caused considerable confusion when the materials were first submitted to Underwriters Laboratories for relative thermal index (RTI) testing. RTI ratings are based on property losses due to oxidation during long-term exposure to elevated temperatures. Typically, a polymer will exhibit a set of baseline properties before aging, which then decline over time when specimens are placed in ovens set at various elevated temperatures. The relationship between aging temperature and the time required to produce a certain loss in initial properties is characterized in an accelerated time frame to produce the RTI values that appear on the UL yellow card.
When the first PPS samples were submitted, they were molded at conditions that did not produce full crystallization. When placed into the ovens crystallization resumed, resulting in anomalous increases in strength and stiffness instead of the expected orderly decline in these properties. Subsequently, the crystallization behavior became better understood and new samples were prepared using the mold temperatures needed to achieve a stable structure. PPS offers some of the highest RTI values available in the industry, with many grades achieving ratings of 200-240°C. The polymer also offers outstanding chemical resistance and is flame retardant without the use of additives. Philips exited the PPS business in 2014, selling the product line to Solvay.
In 1978, the first samples of poly(etheretherketone), or PEEK, were produced at ICI and within three years a full catalog of commercial grades was introduced under the trade name of Victrex. This extended the heat resistance of high-performance semi-crystalline polymers even further. This material, in semi-crystalline form, had an unprecedented melting point of 343°C and a Tg of 143°C. Subsequent innovations in this chemistry produced poly(etherketone), or PEK, with a melting point of 373°C and Tg of 160°C, and culminated in a material with the formidable abbreviation PEKEKK, a polymer with a Tg of 170°C and melting point of 387°C, comparable to that offered by zinc die-cast alloys.
Polyimide-based chemistries were also developed further during this period. Thermosetting polyimide was first created in the lab in 1908 by Marston Bogert and Roemer Renshaw. Commercialization came much later when in 1953 Walter Edwards and Robinson Maxwell at DuPont filed a patent for polyimides based on pyromellitic acid. The product became known as Vespel and still stands as one of the highest performing polymers known. However, the initial chemistries were crosslinked materials. In the late 1970’s and into the late 1980’s the imide chemistry was reimagined in thermoplastic form beyond the realm of Torlon. Perhaps the best-known example of this was polyetherimide (PEI), first developed by GE Plastics (now SABIC), and commercialized as Ultem in 1982 after nearly a decade of development by a team led by J.G. Wirth.
An amorphous polymer, PEI matches the Tg of the high-heat sulfone polymers while offering a fully developed imide structure that does not require the arduous post-mold baking routines needed for polyamide-imide. While the initial PEI materials still had Tg’s that were 65°C below those of PAI, this gap has been narrowed by innovation. Today, it is technologically possible to produce a polymer with a Tg of 310°C (590°F).
However, as with all of these high-performance materials, melt processability within the framework of today’s molding machines becomes the issue. An amorphous polymer with a Tg of 310°C (590°F) is likely to have a minimum processing temperature of 410°C (770°F), very close to the maximum temperature achievable for most molding machines. Consequently, commercial grades for this product line, known commercially as Extem, tend to top out at about 270°C (518°F).
Subsequently, a number of adaptations of polyimide chemistry were adapted to the world of thermoplastic materials. The processing conditions are very demanding, requiring high melt and mold temperatures and high pressures for injection molding. If the material is capable of crystallization, a post-bake routine of several hours may be required to achieve the optimal level of crystallinity. In addition, while melt temperatures must be elevated, these materials can be prone to crosslinking if exposed to processing temperatures for an extended period of time. Therefore, residence times in the melt need to be kept short.
One member of the high-performance family that was developed in this same time period actually has its roots in chemistry developed in the late 19th century, and is worthy of some special treatment. This is the class of materials known as liquid-crystal polymers (LCP). These materials do not appear to fit into the generalized categories of amorphous and semi-crystalline, and they provide some unique characteristics that will be discussed in our next installment.
ABOUT THE AUTHOR: Michael Sepe is an independent materials and processing consultant based in Sedona, Ariz., with clients throughout North America, Europe, and Asia. He has more than 45 years of experience in the plastics industry and assists clients with material selection, designing for manufacturability, process optimization, troubleshooting and failure analysis. Contact: (928) 203-0408 • mike@thematerialanalyst.com
Related Content
What's the Allowable Moisture Content in Nylons? It Depends: Part 2
Operating within guidelines from material suppliers can produce levels of polymer degradation. Get around it with better control over either the temperature of the melt or the barrel residence time.
Read MorePrices of Volume Resins Drop--Except for PE
The downward trajectory appears to be continuing into the first quarter for most resin prices, though PE and possibly PP may remain somewhat stable.
Read MorePrices of Volume Resins Generally Flat or Lower
Exceptions in early March were PP and PS, which moved up solely due to feedstock constraints, along with slight upward movement in PVC and PET.
Read MoreLanxess and DSM Engineering Materials Venture Launched as ‘Envalior’
This new global engineering materials contender combines Lanxess’ high-performance materials business with DSM’s engineering materials business.
Read MoreRead Next
Tracing the History of Polymeric Materials: Polyphenylene Oxide Blends
PPO was a promising new high-performance thermoplastic, but it could be made useful only by mixing it with a humble commodity resin.
Read MoreTracing the History of Polymeric Materials, Part 25: Silicones
The long road to the development of silicone resulted in a chemistry that is remarkably versatile.
Read MoreTracing the History of Polymeric Materials: Silicones
More properly known as siloxanes, silicones are a class of materials where no carbon is present in the polymer backbone.
Read More